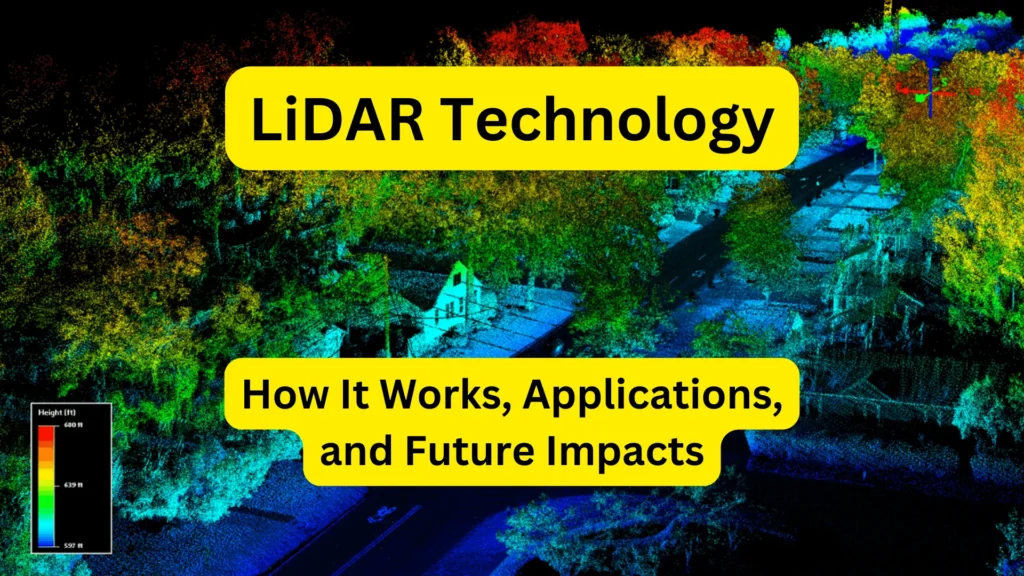
Introduction
Understanding LiDAR
LiDAR, which stands for Light Detection and Ranging, is a cutting-edge remote sensing technology that uses laser pulses to measure distances and create highly detailed, three-dimensional (3D) maps of the environment. By emitting rapid laser pulses and measuring the time it takes for each pulse to bounce back, it provides accurate information about the size, shape, and distance of objects. This information is crucial for creating real-time, 3D representations of surroundings, making it an essential tool in many industries that depend on precision and spatial accuracy.
Unlike traditional methods of surveying and mapping, which can be labor-intensive and time-consuming, LiDAR enables fast, high-resolution data collection over large areas and in various light and weather conditions. This has made LiDAR indispensable in a wide array of applications, from mapping dense forests to guiding autonomous vehicles on busy city streets. Its ability to work in low-light conditions (including nighttime) without compromising accuracy provides a significant advantage over camera-dependent systems. Additionally, its precision has even enabled scientists to reveal hidden archaeological sites under dense vegetation, uncovering previously unknown parts of our history.
Its Role Across Diverse Industries
This technology has revolutionized multiple industries by providing high-precision data for applications previously out of reach. In autonomous vehicles, LiDAR plays a crucial role in safety, creating real-time, 3D maps that help detect obstacles and navigate complex environments, boosting reliability in companies like Tesla and Waymo. Environmental science uses this technology for ecosystem assessment and forest management, mapping areas to track biodiversity and monitor climate change impacts.
Urban planners leverage it to create detailed city maps, aiding in traffic flow analysis, hazard identification, and smart city development. In natural disaster management, this technology assists with floodplain mapping and erosion analysis, helping scientists predict disaster zones and aiding rescue operations with quick, accurate area surveys. Archaeologists have used this technology to reveal hidden ancient sites, such as Mayan cities, preserving historical locations with minimal disruption. As LiDAR advances and becomes more accessible, its applications are set to grow, impacting diverse fields with new insights and innovations.
Understanding the Basics of LiDAR Technology
Defining LiDAR and Its Purpose
LiDAR, an acronym for Light Detection and Ranging, is a highly advanced remote sensing technology designed to measure distances and create precise 3D models of surroundings. It works by emitting rapid laser pulses toward objects and recording the time taken for each pulse to bounce back. This process, known as time-of-flight measurement, is key to generating accurate, high-resolution maps that capture fine details like object size, shape, and distance. Its ability to collect data quickly, even in challenging conditions, has made it indispensable for numerous applications, from autonomous navigation to environmental mapping and beyond.
How LiDAR Works
The basic principle behind this technology involves emitting laser pulses and measuring how long it takes for each pulse to reflect back to the sensor. Here’s a breakdown of the process:
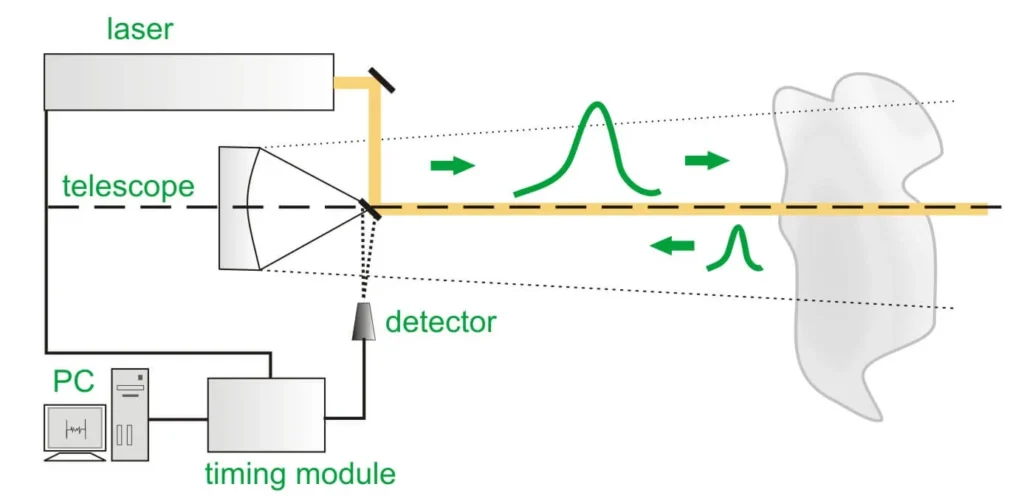
- Emission of Laser Pulses: The LiDAR system emits thousands to millions of laser pulses per second toward the surrounding area. Each pulse travels at the speed of light toward an object or surface.
- Time-of-Flight Measurement: When a laser pulse strikes an object, it reflects back to the LiDAR sensor. The system calculates the time of flight—the time taken for the pulse to return—and uses it to determine the distance between the sensor and the object. This calculation is straightforward yet precise: distance is obtained by multiplying the speed of light by half the time it took for the pulse to make the round trip.
- Creating 3D Maps: By sending and receiving thousands of laser pulses across different angles and directions, it captures comprehensive data on multiple objects and surfaces in its range. The information from each laser pulse is combined to create a highly accurate 3D point cloud—a dense collection of data points that represents the shape, distance, and contours of objects within the environment. These point clouds are processed to form detailed 3D maps, enabling real-time applications like self-driving navigation, topographical mapping, and environmental monitoring.
Key Components of LiDAR Systems
To perform these intricate measurements, a LiDAR system consists of several core components:
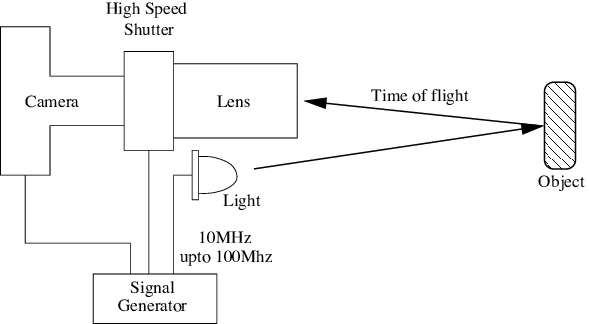
- Laser Source: The laser source is the heart of a LiDAR system, emitting focused beams of light in rapid pulses. Typically, its systems use either infrared or near-infrared wavelengths, which are well-suited for measuring distances. The choice of wavelength can vary depending on the application; for instance, some LiDAR systems in autonomous vehicles may use different wavelengths to improve visibility in various weather conditions.
- Sensor/Detector: The sensor, or detector, is responsible for receiving the reflected laser pulses. It captures the time taken for each pulse to return, enabling the system to calculate distance. High-speed, high-precision detectors are essential for accurate time-of-flight measurement and data collection, especially in applications that require real-time responsiveness, like vehicle navigation.
- GPS (Global Positioning System): The GPS component provides critical location data that enhances the accuracy of the LiDAR measurements. By recording the exact coordinates of the LiDAR system in real time, GPS helps synchronize data with the environment’s geographic location. This is particularly crucial for applications like aerial mapping, where precise positioning is needed to create accurate topographical maps over large areas.
- IMU (Inertial Measurement Unit): The IMU is an advanced sensor that measures the orientation and movement of the LiDAR system. It typically includes accelerometers and gyroscopes to monitor changes in direction, tilt, and speed. In mobile LiDAR applications, such as on vehicles or drones, the IMU plays a vital role in maintaining accuracy despite changes in motion, ensuring that the generated 3D maps remain stable and precise.
Why Its Basic Mechanics Matter
Its effectiveness comes from the precision and efficiency of its components working in unison. The system’s ability to capture millions of data points per second provides unparalleled detail, making it suitable for applications where accuracy is paramount. Its adaptability allows it to be deployed in various environments, from crowded urban landscapes to remote natural settings, without sacrificing data quality. Whether in autonomous driving, environmental studies, or disaster management, understanding how it works, and the purpose of each component reinforces its value as a transformative technology.
Types of LiDAR Systems
This technology come in various forms, each tailored for specific applications. The three primary types—Airborne, Terrestrial, and Mobile/Stationary LiDAR—are optimized for capturing data across different terrains, scales, and project requirements. Here’s an overview of each type and its applications.
Airborne LiDAR
Overview and Purpose
Airborne LiDAR systems are mounted on aircraft, drones, or helicopters to conduct extensive aerial surveys over large areas. This technology is widely used in topographic mapping, forestry, urban planning, coastal monitoring, and environmental management. Airborne LiDAR enables the capture of data over vast expanses, providing valuable insights for projects that require large-scale geographic data.
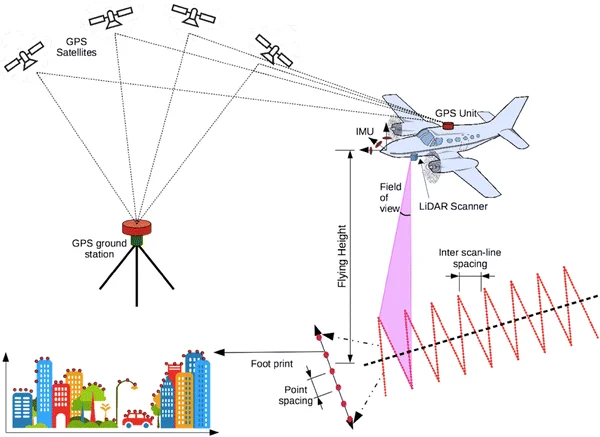
How Airborne LiDAR Works ?
In an airborne LiDAR system, the laser scanner emits rapid pulses toward the ground as the aircraft flies along a predetermined path. The time-of-flight measurement records the time taken for each pulse to reflect back to the sensor, calculating the distance between the scanner and the ground. The combination of the aircraft’s altitude, position (provided by GPS), and the direction of the LiDAR scanner generates a high-resolution, 3D point cloud of the landscape below. By flying at higher altitudes and covering larger areas, airborne LiDAR provides an efficient means to map topography, even in difficult-to-access regions.
Key Applications
Airborne LiDAR is indispensable for several fields:
- Forestry and Vegetation Analysis: By capturing data on tree heights, canopy density, and biomass, airborne LiDAR aids in sustainable forest management and monitoring ecosystem health.
- Coastal Mapping: Airborne LiDAR systems, particularly bathymetric LiDAR, can penetrate water surfaces to map underwater terrain, supporting coastal management and marine conservation efforts.
- Urban Planning and Infrastructure: Airborne LiDAR assists urban planners in modeling landscapes, analyzing terrain, and assessing environmental impacts for new infrastructure projects.
Terrestrial LiDAR
Overview and Purpose
Terrestrial LiDAR systems, often mounted on ground vehicles or tripods, are designed for high-detail, close-range mapping. These systems are ideal for infrastructure mapping, building construction, mining, archaeology, and land survey applications. Terrestrial LiDAR provides precise data at a granular level, capturing details on individual structures or small geographic areas.
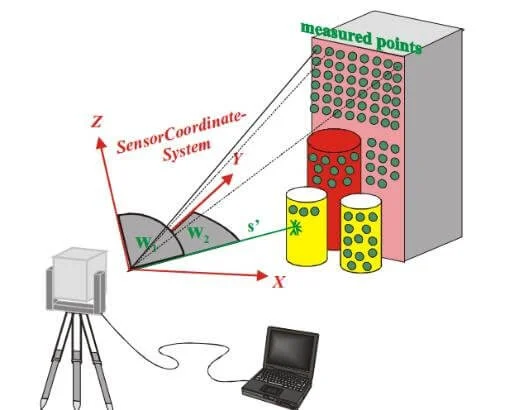
How Terrestrial LiDAR Works?
In terrestrial LiDAR, the scanner is positioned on a fixed ground-based platform, such as a tripod, or mounted on vehicles like trucks or railcars for mobile terrestrial scanning. These systems operate at relatively short distances, allowing for fine detail capture. The LiDAR scanner emits pulses and calculates distances based on time-of-flight measurements, similar to airborne LiDAR but at a lower altitude and within a smaller area. Terrestrial LiDAR provides highly accurate, detailed scans, generating rich data on building facades, road surfaces, bridges, and geological features.
Key Applications
- Infrastructure Inspection: Terrestrial LiDAR can capture structural details and identify potential weaknesses or changes in buildings, bridges, and tunnels, making it vital for maintenance and safety inspections.
- Construction and Engineering: LiDAR is used in construction planning to create accurate models of structures and monitor progress, ensuring that new developments meet design specifications.
- Mining and Geological Mapping: Terrestrial LiDAR helps create topographic maps of mine sites, assisting in the monitoring of slope stability and extraction planning, which enhances safety and efficiency.
Mobile and Stationary LiDAR
Overview and Purpose
Mobile and stationary LiDAR systems offer versatility in how data is captured, with each method serving different survey needs. Mobile LiDAR is mounted on moving vehicles, such as cars, trains, or even boats, for capturing data across large linear or urban areas. This system is particularly useful for mapping road networks, urban environments, and railways. Stationary LiDAR, by contrast, is fixed at a single point and is typically used for close-range, detailed scanning, making it ideal for specific architectural surveys and archaeological documentation.
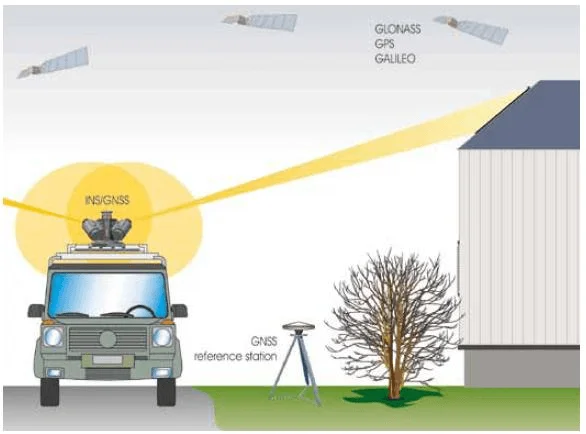
Differences in Usage
- Mobile LiDAR: Mounted on vehicles, mobile LiDAR collects data continuously as the vehicle moves, capturing high-resolution 3D images of the surroundings. This type of LiDAR is used in dynamic environments, allowing rapid data collection over roadways and urban landscapes. Its mobility makes it particularly useful for surveying long stretches of terrain, such as highways or rail tracks.
- Stationary LiDAR: Fixed at one location, stationary LiDAR focuses on detailed scanning within a specific range. This approach is ideal for static, focused scans where high precision is necessary, such as architectural surveys of historical buildings or construction projects. Stationary LiDAR can be deployed on a tripod and set to scan 360 degrees around its location, capturing intricate details that may be missed by mobile systems.
Key Applications
- Transportation and Roadway Analysis (Mobile LiDAR): It is used by transportation agencies to create accurate maps of road conditions, identify maintenance needs, and monitor traffic flow. This data is crucial for urban development and smart city planning.
- Architecture and Historical Preservation (Stationary LiDAR): Stationary Its ability to capture small details makes it a powerful tool for preserving architectural heritage. It enables archaeologists to document ancient structures with precision, creating digital records for future study.
- Utility Mapping (Mobile LiDAR): It is frequently used to survey utility infrastructure such as power lines, pipelines, and communication networks, ensuring efficient monitoring and maintenance in challenging or expansive areas.
Each type of this system—Airborne, Terrestrial, and Mobile/Stationary—offers unique benefits, tailored to specific applications across industries. Airborne LiDAR covers large-scale, aerial mapping projects efficiently; Terrestrial LiDAR captures detailed, ground-based surveys for construction and geology; and Mobile and Stationary LiDAR meet varied demands in transportation, architecture, and infrastructure. As technology progresses, these systems are expected to become more accessible, enabling new applications and continuing to enhance industries that rely on accurate, real-time mapping data.
Major Applications of LiDAR
This technology has revolutionized numerous fields by providing precise, high-resolution data that was previously unattainable. Its applications span from environmental mapping to aiding in autonomous vehicle navigation, showcasing the versatility and importance of this technology across different industries.
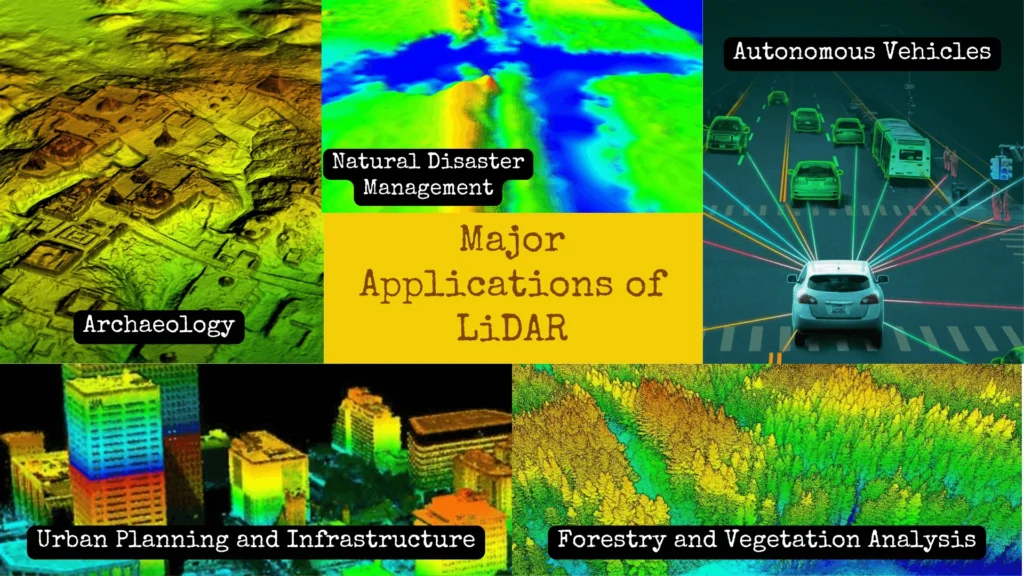
Environmental and Topographical Mapping
Significance of Detailed Topographical Maps
This technology is essential for creating accurate topographical maps, which are invaluable in environmental science and land-use planning. With its ability to capture precise elevation data, LiDAR allows researchers to map the contours of landscapes, valleys, mountains, and waterways with remarkable detail. This data supports geological studies, hydrology, and land management, providing essential information for analyzing soil erosion, water flow, and other environmental factors.
Studying Ecosystems with Precision
In ecosystem research, LiDAR helps scientists monitor and map different habitats, assess biodiversity, and detect changes over time. For example, in conservation areas, LiDAR data assists in identifying human impact on natural landscapes, enabling proactive environmental protection strategies. By combining LiDAR with other data sources like satellite imagery, researchers gain insights into ecosystem dynamics, helping to predict the impact of climate change on vulnerable regions.
Forestry and Vegetation Analysis
Assessing Forest Density and Structure
This technology is instrumental in forestry management, as it provides detailed information on forest density, tree heights, and canopy structure. Through 3D imaging, LiDAR enables foresters to assess tree health, monitor growth patterns, and detect diseases or damage caused by pests. This information allows for better forest management practices, promoting sustainable logging and reforestation.
Monitoring Biodiversity and Biomass
Its ability to analyze forest biomass helps researchers estimate carbon storage, an important factor in climate change studies. By understanding biomass distribution, scientists can evaluate the forest’s role in carbon sequestration, aiding efforts to reduce atmospheric carbon. Additionally, its precision in mapping different layers of forest vegetation support biodiversity assessments by identifying habitat types and enabling targeted conservation of species-rich areas.
Autonomous Vehicles
3D Mapping for Navigation
This technology is a key component in autonomous vehicle navigation systems. It generates real-time, high-resolution 3D maps that allow self-driving cars to understand their environment accurately. By capturing precise spatial data, it helps vehicles navigate complex roads, recognize lanes, and identify boundaries, ensuring smoother operation and greater accuracy in a range of driving conditions.
Obstacle Detection and Safe Autonomous Driving
Its rapid data capture and processing capabilities enable it to detect obstacles, pedestrians, and other vehicles. This feature is crucial for real-time decision-making, as it allows the vehicle to calculate safe paths and react instantly to changes in its surroundings. By providing consistent, high-quality data, it enhances safety, helping to prevent accidents and improve the reliability of autonomous systems.
Urban Planning and Infrastructure
Accurate City Mapping and Construction Planning
In urban planning, it supports the design and development of smart cities by creating highly detailed 3D maps of urban areas. This data assists architects and planners in identifying suitable sites for construction, understanding land use, and planning infrastructure layouts. By enabling precise measurements and location data, it contributes to efficient, well-structured city development.
Infrastructure Maintenance and Management
It is also used in maintaining existing infrastructure, such as bridges, roads, and utilities. Its ability to detect minute shifts or deformations in structures helps engineers monitor the health of these assets, identify potential hazards, and plan maintenance schedules. By ensuring accurate and timely data, it helps cities manage and extend the lifespan of critical infrastructure.
Archaeology
Uncovering Ancient Structures
It has become a powerful tool in archaeology, enabling researchers to discover and map ancient ruins hidden beneath dense vegetation or within rugged landscapes. Unlike traditional survey methods, it can penetrate tree canopies to reveal structures such as ancient temples, pyramids, and fortifications, which are otherwise inaccessible.
Preserving and Documenting Historical Sites
Through detailed mapping, this technology allows archaeologists to document and preserve sites with minimal physical disturbance. This approach is particularly beneficial for fragile historical sites, as it enables thorough documentation while maintaining site integrity. This technology has led to significant discoveries, such as previously unknown Mayan cities in Central America, which have enhanced our understanding of past civilizations.
Natural Disaster Management
Floodplain Analysis and Coastal Erosion Monitoring
This technology is an essential tool in disaster preparedness, particularly for analyzing floodplains and coastal regions. By creating elevation models, LiDAR helps predict areas at risk of flooding, allowing planners to take preventive measures to protect communities. In coastal areas, LiDAR enables the monitoring of erosion patterns, providing data to assess shoreline changes and mitigate damage.
Real-Time Disaster Response Mapping
During and after natural disasters, LiDAR-equipped drones can quickly capture real-time data on affected areas, even those that are difficult to access. This data is used by rescue teams to assess damage, identify safe routes, and locate affected individuals or assets. For example, after a hurricane or earthquake, it scans help relief teams map debris and access critical areas more efficiently, speeding up rescue and recovery efforts.
Its applications across various fields illustrate its transformative impact on data collection, analysis, and decision-making. From aiding in environmental conservation to enhancing urban infrastructure and archaeological research, its precision, adaptability, and efficiency make it an invaluable tool. As This technology continues to evolve, its applications are likely to expand, driving innovation and providing new solutions to complex challenges across multiple industries.
Advantages of LiDAR Technology
Light Detection and Ranging technology stand out as a cutting-edge tool in data collection, mapping, and analysis due to its distinct advantages over traditional surveying methods. By offering high precision, rapid data acquisition, and versatility, it has become invaluable across various industries. Below, we explore the major benefits of it that contribute to its widespread adoption.
Precision and Detail
High-Resolution Data for Complex Analysis
It provides unparalleled accuracy, delivering centimeter-level data that traditional surveying techniques often struggle to match. By emitting thousands of laser pulses per second and measuring the time it takes for each pulse to return, it creates detailed 3D representations of terrain and objects. This capability is particularly advantageous for industries that require intricate mapping, such as construction, urban planning, and environmental science.
Reducing Human Error and Increasing Consistency
Since LiDAR systems automate much of the data collection process, they help reduce human error, ensuring greater consistency and reliability in the results. Surveyors can collect accurate topographical data without needing extensive ground-based measurements, which is particularly useful in regions with complex landscapes, like forests, mountains, or urban environments. This precision also improves predictive models, enabling more informed decision-making.
Rapid Data Acquisition Even Over Large Areas
Efficient Mapping of Large-Scale Regions
It is capable of scanning extensive areas in a fraction of the time required by traditional methods. With aerial LiDAR, for example, large expanses of land can be mapped in a single flight, covering several square kilometers in just a few hours. This speed is particularly valuable for time-sensitive projects, such as disaster response, where rapid assessment of the impacted area is essential for effective planning and resource allocation.
Cost-Effective Over Time
The efficiency of this technology allows it to be cost-effective in many scenarios. Although LiDAR systems may have a high initial setup cost, their ability to gather data quickly and accurately reduces the need for repeated surveys or extensive labor, ultimately saving time and financial resources. This advantage makes LiDAR an ideal choice for large-scale projects, from urban planning to agricultural management.
Works in Various Light Conditions
Independence from Ambient Light
Unlike traditional surveying and photographic methods, this technology is not reliant on natural or artificial light to operate. LiDAR sensors work by emitting laser pulses, which means they can function just as effectively in the dark as they do in daylight. This feature is critical for applications such as search and rescue, where time is of the essence and surveyors may need to work outside standard daylight hours to reach remote or affected areas.
All-Weather Capabilities
Its reliance on laser pulses rather than visual cues allows it to operate effectively in challenging weather conditions, including light fog, mist, and other low-visibility situations. For sectors like aviation, autonomous vehicles, and environmental monitoring, its ability to provide consistent data regardless of lighting or weather conditions is a major advantage, allowing for continuous, reliable data collection.
Non-Invasive
Minimal Impact on Natural or Archaeological Sites
This technology is non-invasive, meaning it can collect data without physically disturbing the area being surveyed. This quality is particularly important in ecological and archaeological applications. For instance, researchers studying endangered ecosystems or protected wildlife habitats can gather data on vegetation density, animal habitats, or terrain features without setting foot in the area. Archaeologists can also use this technology to locate and document ancient structures buried under dense vegetation without excavating or altering the site, preserving it for future research.
Accessibility to Remote and Hazardous Locations
Because it can be deployed aerially, on drones, or on unmanned vehicles, it can reach regions that are challenging or dangerous for humans to access. For example, in forestry or mountainous areas, its sensors can be mounted on aircraft to capture high-resolution data from above, reducing risks to personnel. Similarly, in natural disaster zones, LiDAR-equipped drones or planes can collect data in areas affected by floods, landslides, or earthquakes, providing critical information without endangering lives.
Its advantages—precision, speed, versatility in lighting conditions, and non-invasiveness—are driving its adoption across a range of industries. From enabling the safe navigation of autonomous vehicles to providing conservationists with detailed ecosystem data, this technology has proven indispensable. Its ability to gather accurate, comprehensive, and reliable data with minimal environmental impact ensures that it remains at the forefront of data acquisition technologies. As the technology continues to advance and become more accessible, we can expect Its applications and benefits to expand, supporting innovation and better-informed decision-making across the globe.
Challenges and Limitations of LiDAR
While Light Detection and Ranging technology offers immense benefits and versatility, it also comes with a set of limitations that affect its feasibility and effectiveness in certain contexts. Here, we outline the major challenges associated with it, from high operational costs to data complexity and environmental dependencies, shedding light on why this powerful technology isn’t universally applicable.
Equipment and Data Processing Expenses
Initial Investment and Operational Costs
One of the primary limitations of LiDAR is its high cost, both in terms of equipment and the associated operational expenses. Quality LiDAR systems are typically expensive, with sophisticated airborne units costing hundreds of thousands of dollars. This high price can make LiDAR inaccessible for smaller companies or projects with limited budgets.
Data Processing and Storage Costs
Once data is collected, processing it is a resource-intensive task. LiDAR generates massive datasets that require powerful computing resources, specialized software, and trained personnel to analyze and interpret. The data processing and storage infrastructure can be costly, adding to the total expenditure of a LiDAR project. Given the need for advanced software and skilled data analysts, organizations may also face ongoing operational costs. Although costs are expected to decrease with technological advancements, current expenses remain prohibitive for many applications.
Alternative Solutions May Be More Cost-Effective
In scenarios where budget constraints are a primary concern, traditional surveying methods or less expensive sensors (like photogrammetry) might be more viable, even though they may sacrifice some of the precision and depth offered by LiDAR. Thus, the high costs associated with LiDAR limit its accessibility and adoption in some fields where cost-effective alternatives suffice.
Weather Dependency
Weather Limitations Affecting Laser Return Signals
LiDAR, although resilient in low-light conditions, is sensitive to specific weather conditions. Adverse weather, such as heavy rain, fog, or snow, can interfere with Its laser pulses, weakening or distorting return signals and reducing the accuracy of the data collected. For example, during a rainy day, raindrops can scatter laser pulses, creating noise in the data and affecting the clarity of the mapped area.
Impact on Aerial and Terrestrial LiDAR Scans
For aerial LiDAR, adverse weather can pose even greater challenges. Low cloud cover or heavy fog can obscure a LiDAR system’s view of the ground, making it difficult for airborne units to capture accurate data. Terrestrial LiDAR also faces limitations in areas prone to heavy snowfall or mist, where laser beams may be absorbed or reflected by environmental particles. These conditions may force surveyors to postpone data collection or find alternative methods, potentially delaying projects and increasing costs.
Adaptations for Specific Environments
While technology is advancing to create more resilient sensors, current LiDAR units still face significant limitations in inclement weather. To mitigate these effects, surveys are often scheduled during optimal weather conditions, but this may not be practical or possible in time-sensitive situations like emergency response or seasonal surveys. As a result, the reliance on favorable weather is a significant drawback for LiDAR operations, particularly in geographic regions with frequent or unpredictable weather changes.
Requires Specialized Software and Expertise for Data Interpretation
Large, Complex Datasets
It captures millions of data points per second, resulting in enormous datasets, often referred to as “point clouds,” that represent detailed 3D information. While this data provides unparalleled detail, it is also highly complex and requires advanced processing to convert into usable maps or models. Managing and processing these large datasets can be challenging for organizations that lack the necessary infrastructure or expertise, limiting the potential of LiDAR in these settings.
Need for Specialized Software and Skilled Personnel
Interpreting LiDAR data requires specialized software that can handle and process large point clouds, such as ArcGIS, CloudCompare, and other GIS (Geographic Information Systems) software. These software tools can be costly and complex to operate, necessitating trained professionals who understand both the technical aspects of the software and the nuances of LiDAR data. The need for such expertise often means that organizations must invest in training or hire specialized personnel, adding to operational costs and complicating data workflows.
Difficulty in Analyzing Certain Types of Terrain and Environments
In certain environments, such as dense forests or urban areas with overlapping structures, interpreting its data can be particularly challenging. The laser beams may bounce off multiple objects (e.g., leaves, buildings), creating multiple data points for a single location. This effect, known as “multipath error,” complicates data interpretation and may require additional processing or field validation to ensure accuracy. The data complexity, in such cases, demands an in-depth understanding of This technology and careful calibration, further emphasizing the need for expert handling.
While This technology brings transformative possibilities, its challenges and limitations underscore the importance of context-specific application. High costs, weather dependency, and data complexity can restrict its use in certain scenarios, making it essential for organizations to weigh the benefits against the constraints. However, as This technology continues to evolve, efforts are underway to reduce these limitations, making it more accessible and practical for a wider range of industries.
The Future of LiDAR Technology
The future of This technology is promising, as advancements are rapidly making it more accessible, affordable, and versatile. With innovations in miniaturization, cost efficiency, and expanded applications across various fields, it is poised to play an increasingly central role in technological and societal developments. Additionally, its evolving applications in emerging fields highlight its adaptability and potential as a transformative tool for the future.
Advancements in Miniaturization and Cost Reduction
Miniaturization: Creating Compact and Versatile LiDAR Systems
One of the most notable trends in This technology is the ongoing effort to miniaturize LiDAR systems. Companies are working to develop compact, efficient, and lightweight LiDAR units, allowing for integration into smaller and more portable devices. This trend not only enhances versatility in application but also expands Its potential to be embedded into everyday technology, such as smartphones and wearables, without compromising performance. By reducing size, miniaturization is driving its adoption in a broader array of fields, particularly those requiring lightweight and unobtrusive technology, such as robotics and consumer electronics.
Cost Reduction: Making LiDAR More Affordable
Historically, the high cost of This technology limited its use to specialized industries with significant budgets, like aerospace and defense. However, recent innovations have led to significant reductions in both production and operational costs. For instance, solid-state LiDAR systems, which rely on fewer moving parts, are generally more affordable and robust than traditional mechanical systems. This shift toward cost-effective solutions is making it more accessible to industries such as agriculture, construction, and small-scale urban planning, where budget constraints once posed a barrier. With continuous advancements, LiDAR is expected to become even more affordable, enabling widespread use and further integration into mainstream technology.
Emerging Use Cases
Augmented Reality (AR)
Its ability to provide precise spatial mapping and depth perception makes it an ideal tool for augmented reality applications. By integrating it into AR systems, developers can create more immersive, interactive, and realistic AR experiences, allowing users to interact seamlessly with virtual objects within their physical environments. Applications range from entertainment and gaming to medical training and retail, where LiDAR-enhanced AR experiences can provide significant value.
Precision Agriculture
In agriculture, it is proving invaluable for precision farming practices aimed at optimizing crop production while minimizing environmental impact. Through aerial and terrestrial LiDAR, farmers can create detailed topographical maps of their land, assess soil properties, monitor crop health, and manage resources with greater efficiency. By understanding variations in terrain and vegetation, farmers can implement tailored practices that improve yield, reduce waste, and contribute to sustainable farming efforts. As the demand for food production grows, LiDAR-based precision agriculture is likely to become an integral part of future farming technologies.
Real-Time Urban Traffic Management
It is set to play a crucial role in enhancing urban infrastructure, particularly in real-time traffic management systems. By deploying LiDAR sensors in cities, traffic flow, pedestrian movement, and public transportation systems can be monitored with high accuracy. This data is invaluable for optimizing traffic signals, preventing congestion, and enhancing pedestrian safety. As urban areas worldwide face increasing challenges in managing congestion, pollution, and safety, LiDAR-supported smart city initiatives offer a path toward more sustainable and efficient urban environments.
LiDAR vs. Competing Technologies
Radar vs. LiDAR
Radar (Radio Detection and Ranging) technology is another prominent sensor technology, particularly in automotive and weather applications. Radar operates by emitting radio waves and analyzing their reflections, which makes it effective in diverse weather conditions and for detecting objects at long distances.
However, radar lacks the high-resolution imaging capabilities of LiDAR, making it less effective for applications requiring precise spatial data, such as detailed mapping or object recognition in autonomous vehicles. Conversely, its reliance on light waves provides higher accuracy and resolution but can be impacted by adverse weather. Together, these technologies often complement each other in complex systems, balancing Its precision with radar’s resilience.
Camera-Based Systems vs. LiDAR
Cameras are also widely used for imaging and depth perception, particularly in visual applications such as photography, surveillance, and certain aspects of autonomous driving. While cameras offer color and texture details that LiDAR cannot, they are limited by environmental factors such as lighting conditions and can struggle to accurately perceive depth in 3D space. LiDAR, in contrast, excels at creating accurate 3D maps in any lighting condition, making it more reliable for real-time applications like autonomous navigation. In many systems, especially autonomous vehicles, LiDAR is often used alongside cameras to combine the strengths of both technologies, providing a comprehensive understanding of the environment.
Conclusion & FAQs
This technology has established itself as a pivotal tool in numerous industries, from autonomous driving and urban planning to environmental science and archaeology. Its unparalleled ability to generate accurate, high-resolution spatial data has redefined how organizations approach mapping, analysis, and navigation tasks. Each application of LiDAR brings unique benefits that have expanded our capabilities in understanding and interacting with the world.
As advancements in miniaturization, cost reduction, and integration with complementary technologies continue, it is positioned to drive innovation in both established and emerging industries. With new applications in augmented reality, agriculture, and urban management, its adaptability underscores its potential to become a foundational technology of the future. As accessibility and affordability improve, it is expected to make an even greater impact, shaping developments that will enhance safety, sustainability, and efficiency across multiple sectors. This forward momentum will likely cement Its place as a transformative force in technology, facilitating new insights and possibilities in the years to come.
Frequently Asked Questions (FAQs)
What does LiDAR stand for, and how does it work?
It stands for Light Detection and Ranging. It works by emitting laser pulses towards a target and measuring the time it takes for the light to return. By calculating the time of flight, LiDAR systems can determine the distance to the target and create detailed 3D maps of the environment.
What are the main types of LiDAR systems?
The main types of LiDAR systems include:
1. Airborne LiDAR: Used for aerial mapping and large-scale surveys, often mounted on aircraft.
2. Terrestrial LiDAR: Deployed on the ground, either on vehicles or tripods, to conduct detailed surveys of infrastructure and landscapes.
3. Mobile and Stationary LiDAR: Mobile LiDAR systems are mounted on moving vehicles for dynamic data collection, while stationary systems are fixed at a point for detailed scanning of specific areas.What are some key applications of LiDAR technology?
It has various applications, including:
1. Environmental and Topographical Mapping: Creating detailed maps for ecological studies.
2. Forestry and Vegetation Analysis: Assessing tree height, density, and overall forest health.
3. Autonomous Vehicles: Aiding in navigation, obstacle detection, and ensuring safe driving.
4. Urban Planning and Infrastructure: Supporting city mapping, construction planning, and maintenance of urban infrastructure.
5. Archaeology: Discovering hidden ancient structures beneath vegetation.
6. Natural Disaster Management: Analyzing floodplains and mapping disaster-prone areas for effective response.What are the advantages of using LiDAR technology?
It offers several advantages, including:
1. Precision and Detail: Provides superior accuracy compared to traditional surveying methods.
2. Speed: Capable of rapid data acquisition over large areas.
3. Works in Various Light Conditions: Effective in both day and night conditions, as well as through some weather conditions.
4. Non-Invasive: Allows for studying sensitive or inaccessible areas without causing disturbance.What are the challenges and limitations of LiDAR?
While This technology is powerful, it does face challenges, such as:
1. High Costs: The expense associated with purchasing LiDAR equipment and processing the data can be significant.
2. Weather Dependency: Performance can be affected by adverse weather conditions like rain, snow, and fog.
3. Data Complexity: Requires specialized software and expertise for effective data interpretation and analysis.How is This technology expected to evolve in the future?
The future of This technology includes advancements in miniaturization, making systems smaller and more affordable. Emerging use cases in fields like augmented reality, precision agriculture, and urban traffic management are expected to grow. Additionally, as LiDAR competes with other technologies like radar and camera-based systems, its unique advantages will drive its integration into various applications.
How does LiDAR compare to radar and camera-based systems?
LiDAR offers higher accuracy and detail than radar, making it better for applications requiring precise mapping and object detection. However, radar has advantages in long-distance detection and performance in poor weather. Camera-based systems provide rich visual information but can struggle with depth perception and lighting conditions. Many modern systems combine LiDAR with radar and cameras to leverage the strengths of each technology.
What industries are currently utilizing LiDAR technology?
LiDAR is being utilized across various industries, including:
1. Transportation: For autonomous vehicle navigation.
2. Agriculture: In precision farming for crop monitoring.
3. Environmental Science: For ecosystem assessments and land management.
4. Construction: For infrastructure development and maintenance.
5. Archaeology: To uncover and map historical sites.
6. Disaster Response: For risk assessment and recovery planning.